Among the different existing inverter topologies, the full bridge or the H-bridge inverter topology is considered to be the most efficient and effective. Configuring a full bridge topology could involve too many criticality, however with the advent of full bridge driver ICs these have now become one of the simplest inverters one can build.
What's a Full-Bridge Topology
A full bridge inverter also called an H-bridge inverter, is the most efficient inverter topology which work two wire transformers for delivering the required push-pull oscillating current into the primary. This avoids the use of a 3-wire center tapped transformer which are not very efficient due to their twice the amount of primary winding than a 2-wire transformer
This feature allows the use of smaller transformers and get more power outputs at the same time.Today due to the easy availability of full bridge driver ICs things have become utterly simple and making a full bridge inverter circuit at home has become a kids play.
Here I have explained a full bridge inverter circuit using the full bridge driver IC IRS2453(1)D from International Rectifiers.
The mentioned chip is an outstanding full bridge driver IC as it single handedly takes care of all the major criticality involved with H-bridge topologies through its advanced in-built circuitry.
The assembler simply needs to connect a few handful of components externally for achieving a full fledged, working H-bridge inverter.
The simplicity of the design is evident from the diagram shown below:
Circuit Operation

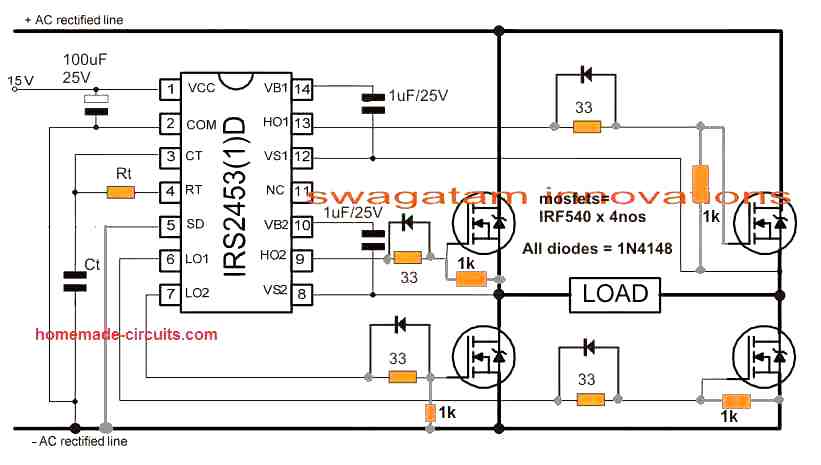
NOTE: Please join the SD pin of the IC with the ground line, if it is not used for the shut down operation.
Pin14 and pin10 are the high side floating supply voltage pinouts of the IC. The 1uF capacitors effectively keep these crucial pinouts a shade higher than the drain voltages of the corresponding mosfets ensuring that the mosfet source potential stays lower than the gate potential for the required conduction of the mosfets.
The gate resistors suppress drain/source surge possibility by preventing sudden conduction of the mosfets.
The diodes across the gate resistors are introduced for quick discharging of the internal gate/drain capacitors during their non-conduction periods for ensuring optimal response from the devices.
The IC IRS2453(1)D is also featured with an in-built oscillator, meaning no external oscillator stage would be required with this chip.
Just a couple of external passive components take care of the frequency for driving the inverter.
Rt and Ct can be calculated for getting the intending 50Hz or 60 Hz frequency outputs over the mosfets.
Important Calculations
Frequency Calculation for IRS2453 Oscillator
The IRS2453 chip uses external components Rt and Ct to set the PWM frequency.
Formula:
f = 1 / (1.453 × Rt × Ct)
- Where:
- f = Switching frequency (Hz)
- Rt = Timing resistor (ohms)
- Ct = Timing capacitor (farads)
Example Calculation:
Let us Assume Rt = 33 kΩ = 33 × 10³ Ω, Ct = 1 µF = 1 × 10⁻⁶ F:
f = 1 / (1.453 × (33 × 10³) × (1 × 10⁻⁶))
f = 1 / (1.453 × 33 × 10⁻³)
f ≈ 20.9 kHz
Thus the switching frequency is approximately 20.9 kHz. So you can adjust Rt and Ct to modify the frequency as needed.
Gate Resistors
The 33ohm resistors at the MOSFET gates limit the inrush current during switching and dampen oscillations.
Power Dissipation in Gate Resistors:
Pgate = Qg × Vgate × f
- Where:
- Qg = Gate charge of the MOSFET (63 nC for IRF540)
- Vgate = Gate drive voltage (10V)
- f = Switching frequency (20.9 kHz)
Substituting values:
Pgate = 63 × 10⁻⁹ × 10 × 20.9 × 10³
Pgate ≈ 0.013 W
Each gate resistor dissipates approximately 13 mW, which is negligible.
Power MOSFET Ratings (IRF540)
Drain-Source Voltage (VDS):
The MOSFETs must withstand the full rectified supply voltage. For a 15V input, VDS(max) must be higher than 15V. The IRF540 has a VDS(max) of 100V, which is adequate.
Current Handling (ID):
Each MOSFET handles half the load current:
ID = Iload / 2
Ensure ID(max) (33A for IRF540) exceeds this value.
Reverse Gate Diodes
The 1N4148 diodes ensures instant gate capacitance discharge for the MOSFETs, which ensures efficient switching response from the MOSFETs.
Reverse Recovery Time:
The recovery time for 1N4148 is 4ns, suitable for high-frequency switching.
Power Dissipation:
Pdiode = Vf × Iload
Where Vf = Forward voltage of the diode (0.7V for 1N4148).
Load Power
The load determines the current through the MOSFETs and resistors.
Load Current (Iload):
Iload = Pload / Vsupply
For Pload = 50 W and Vsupply = 15V:
Iload = 50 / 15 = 3.33 A
Power Dissipation in MOSFETs:
PMOSFET = ID² × RDS(on)
For IRF540, RDS(on) = 0.044 Ω:
PMOSFET = (3.33 / 2)² × 0.044 = 0.122 W per MOSFET.
Capacitor Selection
Input Capacitor (100 µF/25V):
Filters the rectified AC and smooths the supply voltage. The ripple current rating should exceed the load current (Iload).
Bootstrap Capacitors (1 µF/25V):
These provide gate drive voltage for the high-side MOSFETs. Ensure the value can handle the gate charge (Qg) of the MOSFETs.
High Voltage Feature
Another interesting feature of this IC is its ability to handle very high voltages upto 600V making it perfectly applicable for transformeless inverters or compact ferrite inverter circuits.
As can be seen in the given diagram, if an externally accessible 330V DC is applied across the "+/- AC rectified lines", the configuration instantly becomes a transformerless inverter wherein any intended load can be connected directly across the points marked as "load".
Alternatively if an ordinary step-down transformer is used, the primary winding can be connected across the points marked as "load". In this case the "+AC rectified line" can be joined with pin#1 of the IC and terminated commonly to the battery (+) of the inverter.
If a battery higher than 15V is used, the "+AC rectified line" should be connected directly with the battery positive while pin#1 should be applied with a stepped down regulated 12V from the battery source using IC 7812.
Although the below shown design looks too easy to construct, the layout requires some strict guidelines to be followed, you may refer to the post for ensuring correct protection measures for proposed simple full bridge inverter circuit.
Simple H-Bridge or Full Bridge Inverter using two Half-Bridge IC IR2110
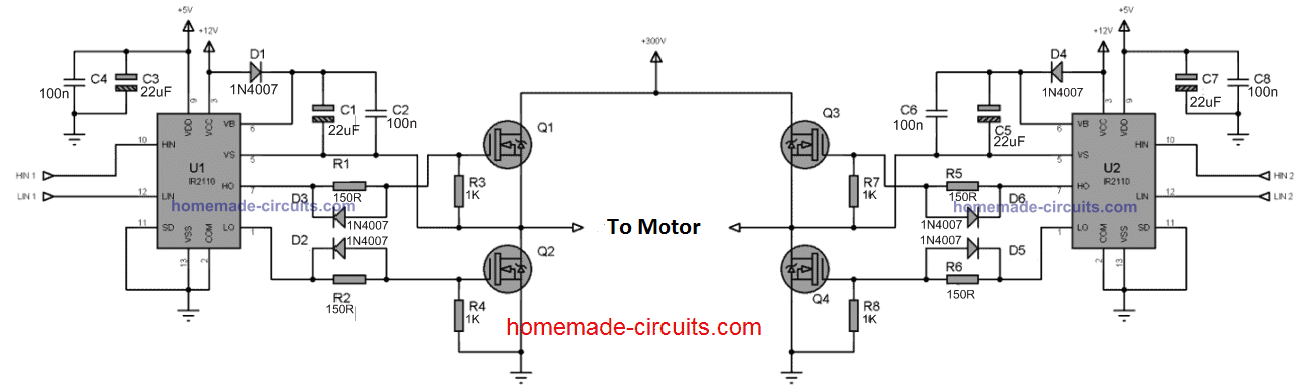
The diagram above shows how to implement an effective full bridge square wave inverter design using a couple of half bridge ICs IR2110.
The ICs are full fledged half bridge drivers equipped with the required bootstrapping capacitor network for driving the high side mosfets, and a dead-time feature to ensure 100% safety for the mosfet conduction.
The ICs work by alternately switching the Q1/Q2 and Q3/Q4 mosfets in tandem, such that at any occasion when Q1 is ON, Q2 and Q3 are completely switched OF and vice versa.
The IC is able to create the above precise switching in response to the timed signals at their HIN and LIN inputs.
These four inputs needs to be triggered to ensure that at any instant HIN1 and LIN2 are switched ON simultaneously while HIN2 and LIN1 are switched OFF, and vice versa. This is done at twice the rate of the inverter output frequency. Meaning if the inverter output is required to be 50Hz, the HIN/LIN inputs should be oscillated at 100Hz rate and so on.
Oscillator Circuit
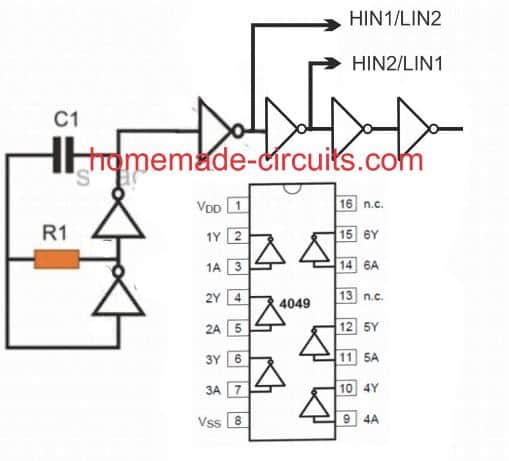
This is an oscillator circuit which is optimized for triggering the HIN/LIN inputs of the above explained full-bridge inverter circuit.
A single 4049 IC is used for generating the required frequency and also for isolating the alternating input feeds for the inverter ICs.
C1 and R1 determine the frequency required for oscillating the half bridge devices and could be calculated using the following formula:
f = 1 /1.2RC
Alternatively, the values could be achieved through some trial and error.
Discrete Full Bridge Inverter using Transistor
So far we have studied a full bridge inverter topologies using specialized ICs, however the same could be built using discrete parts such transistors and capacitors, and without depending on ICs.
A simple diagram can be seen below:
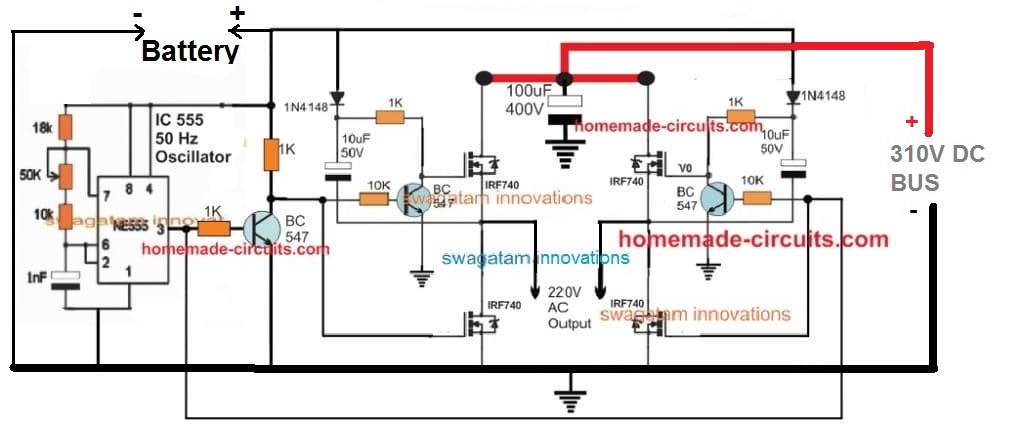
Have Questions? Please Leave a Comment. I have answered over 50,000. Kindly ensure the comments are related to the above topic.
El tl494 para que sirve
tl494 is an oscillator IC.
Gracias por ayudarme a mejorar las experiencia en electrónica
Porque los diodos de salida del inversores se calientan
which diodes are you referring to exactly?
hello sir i hope you good,, i have talk with you about full h-bridge solar inverter 5.5kw and volatge from the solar is 490v and i am using SPW47N60C3 mosfet and 10 mosfet on each channel all mosfets i will use 40 mosfets ….10 on each channel i will use 2 IR2110 ic and cd4047 for pwm for 2 IR2110 ic to drive mosfets to make it full h-bridge
i have a question and thanks you for your time.
how much current the circuit need for ics to feed gate mosfets without any problem and what is the best voltage?
Thank you Sayed,
The MOSFET gates are high impedance inputs so the current does not matter, the gates can work with microamperes also, so nothing to worry about the current. The best voltage is 12V.
The ICs might need 5 to 10mA for operating correctly.
okay i want ask you again and i am sorry for taking from you time.sir
i will make circut without transformer to feed the ics and gates of mosfets
12v 150 ma it will handle the gates and ics?
question two the gates takes power from the 2 IR2110 ics and cd4047 right?
No problem Sayed,
Yes 12V 150mA will more than sufficient for the ICs and the MOSFET gates.
Your second question is also correct. However remember that the HIN pin of one IR2110 IC must connect with the LIN pin of the other IR2110 IC, and vice versa.
make full h-bridge solar inverter 5kw and i am using irfp460 amd 10 mosfet on each channel all mosfets i will use 40 mosfets ….10 on each channel i will use 2 IR2110 ic to drive mosfets to make it full h-bridge
i have some questions and thanks you for your time.
1. can i use tl494 as an oscillator for 50hz for 2 ic ir2110?
2. what is the best resistor to add on gates mosfets on each channel?
Yes, those MOSFETs can be used, provided your solar input voltage is above 200V DC.
TL494 cannot be used to generate 50Hz frequency. You can use 4060 iC instead, or the one explained in the above article.
For 50Hz you can use any gate resistor between 10 ohm and 50 ohm 1/4 watt.
thanks for your answer sir,
but in ic4060 can i add feedback to adjust votlage automaticaly ??
and i asking i should add resisiter on pwm signals coming from ic4060 to 2ic ir2110 like mosfets gates ?
and thanks for your time sir
Hi Rony, yes a feedback can be added to a 4060 based inverter circuit, but a 4060 will require another NPN BJT for inverting the output for the HIN, LIN inputs.
The outputs from the 4060 IC to the HIN, LIN inputs of the H-bridge will not require any resistors, they can be connected directly.
However I think the oscillator using the 4049 IC, as given in the article, is even better since it won’t require an external NPN BJT, and a feedback can also be added to this 4049 circuit.
okay so the 4049 IC is better and i can add feedback for this inverter without any problem?
and if you have a circuit of ic4049 and how add feedback i will be greatfull and hanks for your time
You can configure the feedback in the following manner: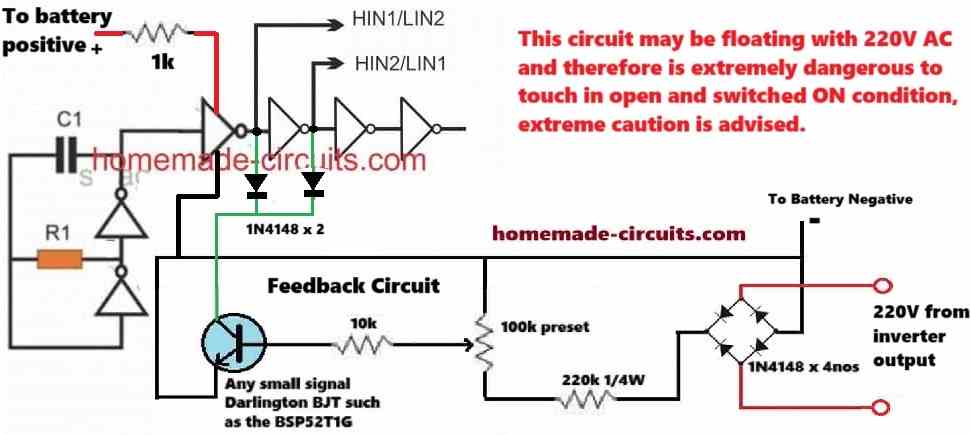
hello if i use cd4047 ic instead of cd4049 ic is ok?
i mean cd 4047 is ok like cd4049?
and thanks for your support and help me and other beginers
Yes, that’s a good choice, you can use the two outputs of the 4047 IC to feed the HIN, LIN inputs of the H-bridge IC.
okay sir thanks alot
but for confirm as you know i will add feedback to adjut voltage automatic so it will working well and i should not add another NPN BJT for inverting the output for the HIN, LIN inputs ??
Hi Rony,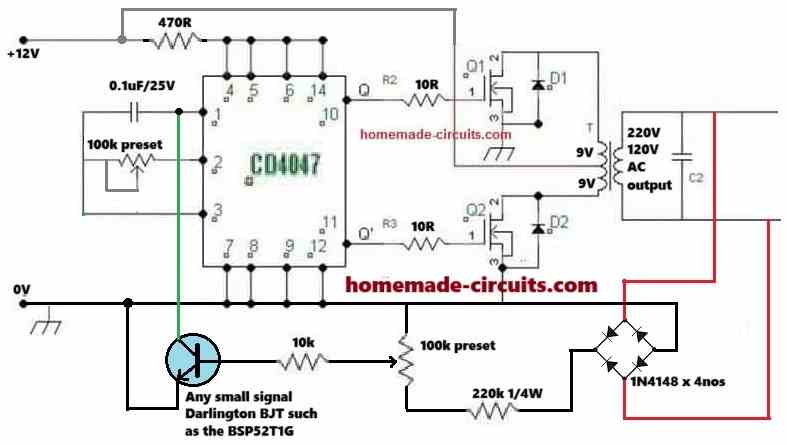
you can add the feedback to the 4047 IC in the following manner:
There’s no need of another NPN BJJT for the HIN, LIN inputs, you can directly connect the two outputs from pin#10 and pin#11 of the 4047 IC to feed the HIN, LIN inputs of the H-bridge iCs.
hello sir welcome again and thanks for your great advices
i have told you At the beginning of the conversation((make full h-bridge solar inverter 5kw and i am using irfp460 amd 10 mosfet on each channel all mosfets))
can i use SPW47N60C3 mosfet instead of irfp460 in the circuit??
Rony, The SPW47N60C3 VDS is rated at 650V, so if your inverter output is rated to work with a voltage above 150V and below 400V then this MOSFET will be good, you can use it.
okay sir you answer me with this (Rony, The SPW47N60C3 VDS is rated at 650V, so if your inverter output is rated to work with a voltage above 150V and below 400V then this MOSFET will be good, you can use it.)
if i used solar panals around 450 volatge at open circuit it willl work fine or i will face problem?
i made a reply on old message because thr last messge did not give me option to reply and thanks again
Yes, if your inverter is rated to work between 400V and 500V then the 650V VDS rated MOSFET will be good enough. You can go ahead with it, no problems!
The comment threads can handle a maximum of 10 replies, after 10 replies the reply option will not be available, then you have to create a new thread…
hello i want to ask you sir , i want to make full h-bridge solar inverter 5kw and i am using irfp460 amd 10 mosfet on each channel all mosfets i will use 40 mosfets ….10 on each channel i will use 2 IR2110 ic to drive mosfets to make it full h-bridge i want to ask you it will work 100% duty cycle right and it is okay?
Yes, that will work, but the duty cycle cannot be 100%, it should lower than 50%.
but i need duty cycle be 100% because voltage of solar 400v i need to get full power can you suggest i will use feed back on it
Yes you will get around 100% power output from the inverter output. By 50% duty cycle I mean to say the output from the ICs will 50% ON and 50% OFF, as we have in a square waveform.
okay sir , are you have any video or article can help me to add feedback on this circuit and make frequency 50hz
Sure, for feedback control you can implement the second concept which I have explained in the following article:
https://www.homemade-circuits.com/load-independentoutput-corrected/
50 Hz frequency will be controlled by the input oscillator, feeding the square waves to the IR2110 ICs.
Good morning Sir,
Thank you for the reply,
If I add short-circuit protection using comparator lm358 then what will be the value of shunt resistor (current sensing resistor) for 3kw load and the value of vds and IDs of MOSFET for pussing the ground to the circuit ?
Good Morning Rinku,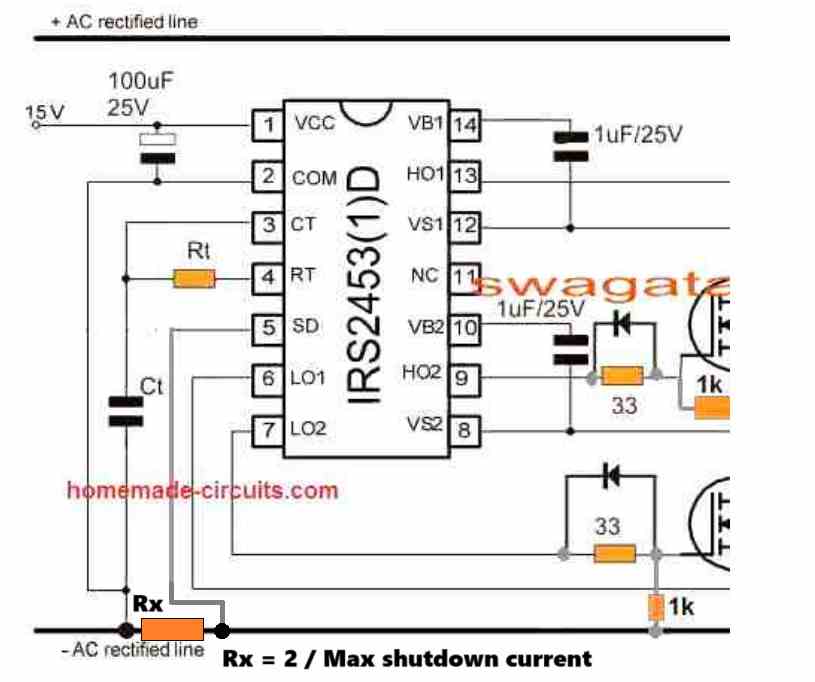
You can configure the shutdown pin SD of the IC in the following manner:
Remember, this will latch up the circuit, meaning if an over-current is detected the IC will shutdown permanently, until power is switched OFF and ON again.
Good morning Sir,
For the scenario of IRS2453 the high side HO1 &HO2 gives 50 hz square wave and we punching 2khz or higher through LO1 &LO2 ,does this make sign wave as both the high side switch received 50Hz. If we give one high side HO2 with 2kHz and LO1 with 50Hz and vice versa then proper punching happens to create sign wave .plz give insight on this as I have seen esg002 card works on this principle
Sir, my simple question is we are giving only low side spwm signal so does it make signwave properly as my mosfet blasts.plz help
Regards
Rinku
Good Morning Rinku,
Applying SPWM to the high-side MOSFETs, which require bootstrapping for proper operation, could be problematic.
The low-side MOSFETs, however, are simpler to control with SPWM because they connect directly to ground. By controlling the low-side MOSFETs with SPWM, the load current will follow the same pattern.
To make sine wave you just need to chop the low side MOSFETs.
In your design are you following the principles explained in the following article:
https://www.homemade-circuits.com/mosfet-protection-basics-explained-is/
If you chop the low side MOSFETs with SPWM the output will also SPWM because the low side is in series with the high side MOSFET.
Remember for iron core transformer the SPWM frequency must not exceed 300Hz.
Dear Sir,
I am using this circuit for transformer less inverter so I have to give 800Hz to 2500Hz spwm signal to the low side MOSFET, Your opinion plz
Rinku, which circuit exactly, please provide the link?
Please try with low frequency first, 300 Hz from the 555 IC.
And change the 100 ohm gate resistors with 1k.
Make sure you have the 1N4148 diodes connected parallel to all the gate resistors.
Dear Sir,
Is the 1k resistor to be added to all the gates or only lowside gate to be connected with?
Hello Rinku, it is for the low side MOSFETs only.
Please also add 10k resistor directly across the gate/source of all the MOSFETs.
Two half bridge IC using ir2110
yes you can use 22uF/25V for all those capacitors.
Pls what is the value of c1,c3,c5,c7 or can I used a 16v or 25v by 22uf in all
which circuit diagram are you referring to?
Pls if am to power the circuitry of ir2110 with a 24v battery for inverter apart from protecting the MOSFET and the gate are their other things to change or value increase
You do not need any changes for operating the IR2110 inverter with a 24V, just make sure the IC supply pins get the recommended 12V and 5V supplies.
Hi Sir,
Can I use TLP250H OR HCPL3150 as gate driver to protect the IRS2453 FOR both high and low side
Hi Rinku, I don’t think those opto-couplers can be used for the high-side MOSFETs for the above explained full bridge inverters, and moreover, it is simply not required because the above circuits are already well protected from all adverse situations.
Pls my question is about the +5vdd and +12vcc of ir2110 how do I connect the two to 24v battery should I separate the two using two regulator lm7805 and 7812 please this is my earlier questions
Yes, you can do that!
Pls am using 4047ic for my PWM signal for the h bridge, how can I connect +5vdd and +12vcc of ir2110 to my 12v regulator am using one voltage regulator for my 4047ic
If you ae using 12V for the VCC pin of the IR2110, you can use the same 12V for the 4047 IC also, a regulator may not be required. If you want to regulate the 4047 supply, you can do it by supplying the 12V to the 4047 IC through a 1k resistor and then adding a 12V zener diode across the 4047 supply terminals.
How can this circuit be powered by a 24v battery and IC 4047
In the first diagram, you can use +24V DC at the AC rectified line attached with the High side MOSFET drains. Use resistor and a 15V zener diode to drop the voltage the IC VCC supply.
I am looking for a way to drive a piezo device for a hydrophone at 200V P-P bipolar anywhere from 15KHz to 20KHz. My supply voltage is a 3.7V 2500mAh battery. I am looking at your dual IR2110 as a possible candidate but I am desperately trying to keep circuitry size and weight to a minimum. I very much appreciate your generosity of how you are helping folks like me and welcome any and all advice you might be able to afford me. Thank you!
Do you want to convert your 3.7V to 200V, or do you have the 200V separately?
However, the IC IR2110 and the associated MOSFETs might not work correctly with a 3.7V supply.
We make 117.6v battery packs for EV’s. Is it possible to purchase a converter to run a refrigerator during a power outage?
Sorry, we don’t sell electronic items, however you can try building one of the inverter designs suitable for your purpose…I can suggest one if you want.
OK, sounds good. We have some other challenges if you are up to it. One is we need a lightweight remote disconnect for 117.6 DC volts 100 amps that doesn’t use an active coil that stays energized – just 12v switch control on and off. Another is a BMS that can balance and supply current as it is receiving a charging current. Plus a few more.
Regarding the first requirement please elaborate a bit…is the 12V switch supposed to be a remote controlled relay?
Yes
What type of remote control, RF or IR?
Either one that works. By wire is best. The location is out of reach.
By wire will do? Meaning it does not need to be wireless? In that case you simply need to connect a 12 V DC to a 12 V relay with 125 amp contacts, that’s all.
Hello, excellent work, I would like to experiment with the diagram of the square wave inverter (the one with discrete components) and I was wondering if it would be useful to use an H bridge but with IGBTs, taking advantage of the high voltage that these support. Could you tell me if theoretically it would work well or if something should be added to the diagram.
Thanks yeiler, glad you liked the post!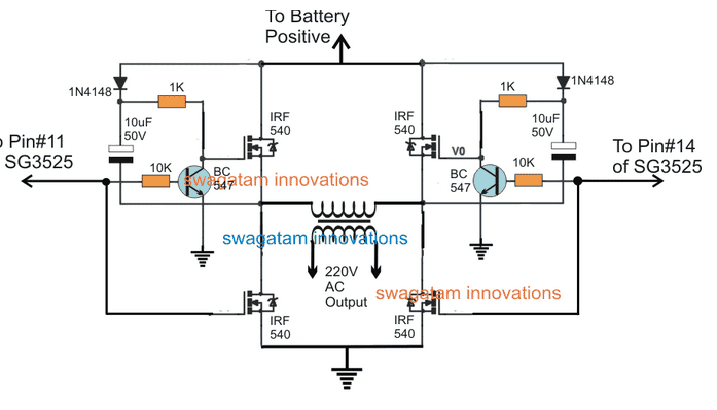
I would suggest you to try the basic design first using mosfets and 12V supply. If it works OK for you then you can upgrade it with high voltage and IGBTs:
Hi Swagatam,
Congrats! Your web page is cool.
I am looking for good recomendation concerning 1000 VA converter 230V/50Hz to 110V/50Hz.
Do you think using IRS2453 could be good idea?
I have the EG002 (EG8010) in my mind.What would be your idea?
Thank you in advance.
Andy
Hi Andrzej, I think the EG002 can be an easier option, but I can’t figure out how this can be used to convert 220V to 110V? If you use PWM then the average voltage will reduce to 110V but the peak will still remain 220V. A transformer is perhaps the ideal option for converting 220V to 110V.
Hi Swagatam,
Thank you so much for your prompt answer. Regarding PWM you are absolutely right.
I am looking for the solution without transformer – step down converter.
Can you suggest one?
Output voltage could be distorted, no need of ideal sinus.
I appreciate your support very much.
Hi Andrzej,
For 220V the peak voltage is 310V and for 110V it should be around 160V
But without a transformer the peak will remain 310V for 110V sinewave, which is not good. I have no idea how this can reduced to 160V without a transformer?
So I think without a transformer reducing the peak to 160 V may not be possible, or maybe I do not know how to do it.
Hi Swagatam,
Thank you very much for the clarification.
Best regards
Andrzej
I have installed a global inverter circuit using discrete transistors. When I test with 12VDC supply from battery the circuit works fine. Output 11.8VDC, frequency 50Hz. However, when I increase the supply voltage to 42 VDC from behind the diode bridge of an iron core transformer. Then immediately a BC546 (high side) was short-circuited. The corresponding mosfet is also shorted. Can you give advice on this case. Thank you very much.
I think it is due to high voltage spikes which may be destroying the transistors. Try installing 100 V or 150 V rated transistors and see if that helps or not.
Thank you for your quick response chings. Can I replace it with the corresponding type ?
I used Mosfet IRF740, transistor BC547.
Yes, you can replace them with other variants rated at 100V or above.
Bonjour Monsieur,
I am trying to design a full bridge using two IR2153 (instead of IR2110 in your schématics).
I want it to be driven by a single Hall effect IC to replace the RC resonator of your suggested oscillator circuit which triggers the IR21xx ICs.
The purpose is when the Hall IC is High, current in the load will flow one way and when low, the other way.
Any idea ?
Thanks
Hello Lhopiteau,
The IR2153 has a built in oscillator, so it cannot be driven by an external signal from a hall effect sensor.
Hello sir
I’m Jose a freshman in electronic engineering.
I have a project at home which I basically want to achieve inversion of 230vdc 60 amps 50hz to 230vac 60 amps 50hz pure sinewave.
I therefore inquire if I can use a thyristor or how can I go making such inverter with which components specifically?
Hello Jose,
Unfortunately a thyristor cannot be used for switching a DC supply. You will have to implement one of the full bridge designs explained in the above article.
You can first try the basic square wave full bridge inverter as explained above, if you succeed with it then we can proceed with the sine wave integration.
Hello sir,
I’ve followed and built the second schematic which uses IC 555.
I therefore request if I can apply the 235VDC 60 amps on the input labeled battery or should I not exceed a certain Voltage limit?
You should strictly apply only 12V across the points labeled as battery, otherwise the IC 555 will burn.
Hello sir,
I’ve just implemented the other H-bridge with 555IC but I’m yet to connect a power source.
So,my question is should I apply at the input labeled battery the 230VDC 60amps or should be a smaller voltage than the 230VDC?
Thank you sir.
Hello Jose,
I think you should first try it with a lower DC voltage, maybe a 12V or 24V, and if it works only then go for the 220V DC.
good work, what is that some wave inverter all about
Hello, excuse me
I am a final year student and I have to finish this project I am sending you the schematic of the circuit and please guide me what kind of MOSFET to use so that the circuit works well and is not expensive.
Also HIN-LIN waveforms
What do you think I should remove or add in the schematic?
Any help, I thank you
sorry for english
https://freeimage.host/i/gUtZE7
https://freeimage.host/i/grbGDb
From the diagram it seems you are trying to use a transformer as the load across the H-bridge circuit. The connections look OK to me.
I hope the mosfets and the transformer winding ratings are in accordance with the VDD supply
Hello
I measured the gate input voltage of the MOSFETs and it was about 4.3 volts.
Can this low voltage cause MOSFETs to heat up?
To increase the amplitude of my PWM pulses.
have you any recommendation
Yes 4.3V will normally heat up a mosfet since most mosfets are rated to work with a minimum of 9V gate voltage.
However your readings might be wrong, because the output of an H-bridge IC can be in a form of a high frequency, which may not allow you to measure the gate voltage correctly.
PWM amplitude is not related to the output voltage of the H bridge, the Vcc voltage is related to the output voltage from the H bridge. The Vcc should be ideally around 12 to 15V. The PWM peak voltage should not be higher than the Vcc voltage.
In your case as I mentioned earlier, a 900V mosfet can never be used with 4500V supply.
If we use a voltage boosting transformer, should we still use MOSFETs with a voltage of 6000 volts?
According to the design, the primary voltage is about 300 volts, which is converted to 4500 volts in the secondary.
According to the above information, is it correct to choose 2SK3878, ID=9A, VDSS=900V MOSFET?
If you are using the 4500 V across the drain/source of the mosfets then the mosfets will need to be rated at over 5000 V or 6000 V. If you are using 300 V across drain/source of the mosfets then your existing 900 V mosfet will be OK.
Thank you for your response
This internal dead time (IR 2113) is enabled, or needs to be enabled.
In which part of the datasheet is it referred to?
Unfortunately it is not mentioned in the datasheet but it is an understood fact that dead time will be existing in the IC by default. If suppose the IC does not provide a dead time internally then the IC will simply not work and it will keep blowing the mosfets which I don’t think the manufacturers of the IC will allow to happen.
This IC is popularly used for making inverters and in no circuit diagram an external dead time has been seen so far.
The main thing is that the mosfets must be rated much higher than the load supply specifications. If the mosfet rating is less than the input supply then it will keep blowing.
No, the output voltage is completely DC and the output ground is connected to the MOSFET ground with a 22 nanofarad capacitor
If the mosfet supply is DC then it is fine, but make sure mosfet VDSS rating is higher than the supply voltage used.
For MOSFETs, I used 2sk3878 with 900V voltage and 9A current.
In this circuit, the output voltage is 4500 volts with a current of 0.005 amperes
900 V can never handle 4500 V supply. The MOSFETs must be rated at least at 6000 V. Meaning the VDSS should be equal to 6000 V minimum.
Yes, the output voltage is DC
This is the circuit diagram for creating dead time.
which is designed for a delay time of 1 microsecond
https://freeimage.host/i/UteuFp
Do you think it is well designed?
The IR 2113 already has a dead time built-in, so there’s no need to create an external dead time.
Thanks for your tips
I create a voltage of 4500 volts using a step-up isolation transformer.
The primary voltage of the two ends is around 300 volts, which the transformer converts to 4500 volts.
How can I send you the map?
If you have used the second diagram from the above article then I can understand the design, no need to send the diagram. However if you want to show the diagram, you can upload it to any “free image hosting site online” and provide the link to me here.
I guess you have used 4500V DC across the drain and the ground of the mosfets. Please Remember the 4500V must be a DC, not AC, otherwise the mosfets will burn.
In that case you will have to upgrade all the mosfets and the capacitors to high voltage rating as suggested in my previous comment.
Thank you for asking this question.
Are you sure the output is 4500 voltage?
Which mosfets did you use? For 4500V output the mosfets must be rated at minimum 5000 to 6000V
Hello
Thank you in advance for sharing your experience.
I am building an H bridge.
80 kHz switching frequency.
The output voltage is 4500 volts.
The driver used is IR 2113.
Designed for a dead time of 1 microsecond.
Problem:
When I test the driver without connecting to the MOSFETs, everything is correct and there is no problem.
But when I connect HIN1/LIN2 MOSFETs Q1 and Q4 and HIN2/LIN1 to MOSFETs Q2 and Q3 and connect the drain voltage, one of the MOSFETs and two IC drivers burn.
Please help me
At 4500V the risks can be very high.
You will have use mosfets that are rated at 6000 V, and the bootstrapping capacitor must be rated at 10000 V. You must have reverse diodes rated at 10kv across mosfet drain/source.
The Vcc pin of the IC must be protected with a 1K series resistor, a filter capacitor, and a 12V zener diode.
The mosfets must be connected as close as possible to the IC. The +/- 4500V DC bus line must have a non polar capacitor rated at 10uF / 10000 V.
Also remember that the HIN and LIN polarities must be perfectly opposite while switching. Meaning when HIN is high, LIN should be LOW and vice versa.
Another thing is that, if you are two IR2113 ICs, the HIN pin of one IC should be connected with the LIN pin of the other IC, so that the mosfest are able to switch diagonally.
The second question is where the last two Gates NOT are connected in the 4049 circuit
Those two gates are extra gates in the IC, they are not used. Just connect them as they are shown in the diagram.
Hello Boss, I built the IRS2453 circuit and it worked but where I have issues is, when I connect 1k across the gate/source of each MOSFET, the output voltage is around 144v instead of 220v but when I remove the resistors, it gives me 220v. Transformer is 12v/220v. Pls what could be reason for this?
That’s great Patrick,
1K should have been fine, I am not sure why you are facing this issue. You can try replacing the 1K with 4k7 or 10K resistors and see how it works.
Thanks for your quick response. I have used 10k yet i can’t get 220v. With the resistors at the gate/source pin, I realised about 4v on the load(where the transformer is expected). Don’t know if the 1uf used for bootstrapping should be made higher, perhaps that might solve the issues.
In that case I think you can eliminate the gate/source resistor for the high side mosfets. However keeping it connected on the low side mosfets won’t have any problems.
Thanks Boss, I’ll try this one and give you a feedback soonest. I sincerely appreciate your good contributions.
Sure thing! Let me know if you have any further questions.
dear Swagatam;
I was able to bridge h with help from this site. thanks but i’m trying to make my circuit better. I want to add IR2110. My circuit is running at 20khz. What are your thoughts? frequency generator ir2153 or (sg3525) hin1 and hin2 connections should be. and your thoughts.
Thank you Mesut, I am glad you could build the H bridge circuit.
you can use the frequency generator circuit which is shown in the 3rd diagram using 4049. Using SG3525 or IR2153 can be quite complex.
in the picture inspect (mosfet gate R5 and R7) does it act as a voltage divider? then mosfet gate (10.4348volt) then R5=47ohm R7=10kohm mosfet gate (11.94volt) wouldn’t the mosfet gate be nicer? Can I use D5,D6,D2,D3 ST4148 diode? and what is the meaning of this diode?
I finished this circuit with IR2153 and IR2111 and I am making my final preparations to try it. I have to calculate the resistance values well, I don’t have much luck if I burn it.
sorry for english.
The gate/source resistors are to increase working efficiency of the mosfets, so that their gate capacitance discharges as quickly as possible during OFF times and the mosfet generates less heat. The diodes also do the same thing, discharges the gate capacitance of the mosfets quickly during OFF times
yes you can use 1N4148 diodes.
Full bridge to drive DC motor ,DIS,about dead time,then pwm1,pwm2,to drive,one way,or back,direction,what,s happen and circuitry how to drive by logic signals?,, ,,,no answer
Hello, thank you for your scientific help. Please help me by using irs2453 and ir2110 for induction furnace circuit. Thank you.
Hi, you can perhaps try the following concept:
https://www.homemade-circuits.com/induction-heater-circuit-using-igbt/
Sayın SWAGATAM.Transistor ve 555’entegreyle yapılan H köprü inverteri yaptım fakat devre çalışmıyor sorun nerede? düzeltip bildirir misiniz? Teşekkür ederim
Hello Sezgin, without checking your circuit practically, it can be difficult to understand the fault.
I am waiting your working circuit schematics please
All the above circuits are tested and verified designs. They have been tested by many of the readers.
Can i also use two of this dual IGBT?
FF150R12RT4
IGBTs can be replaced for MOSFETs, but I am not sure about the specific number you have mentioned.
Thank you very much sir ????
My pleasure!
Hello sir, according to the final circuit do I need to use resistors to discharge the gate capacity?
Hello Buddhi, resistors are not required, gate capacitance is discharged by the BC547 transistors
Me encantan esos proyectos me gustaría hacerle una consulta espero vea mi comentario
hi, can I use this method for product frequency above 1MHz?
I need help troubleshooting the half bridge inverter using a IR2110. Can you help me out?
Hi, I would like to use a 4049 to make a oscillator and use a IR2110 to make a half bridge for creating a wire power transfer circuit. However, I just have one rectifier, and cannot fully apply your circuit. How can I modify your circuit above, so I can make my circuit work? Thank you!!
Hi, you can use a single diode rectified voltage, by including a high value filter capacitor across the rectified lines. The capacitor could e in the order of 500uF/400V
Sir,
Mosfets connected to pins 13 and 8 do not switch ON at all. accordingly no output power.
do you have any comments.
Thanks
Please check the frequency across the Ct capacitor, if you get a frequency there, then your IC is oscillating and the MOSFET may be faulty, if no frequency then your IC is faulty or some other part/connections are faulty…
Any oscilloscope can quickly indicate the working of the IC.
Sir,
RE:Simple full bridge inverter using driver IRS2453dpdf
I just made similar circuit using 20VDC battery with voltage divider (16V power tge driver) but l used mosfet FQP16N25 instead of IRF540.
at the end the circuit not producing AC power at all.
l am not sure what is the problem, is it the mosfet type or what.
I appreciate your advise. Thanks
Sam, did you connect the shut down SD pin to ground. Please check out the revised diagram, showing the SD pin connected with ground, and 1k resistors connected across the gate/source of all the MOSFETs
Yes sd pin connected to the ground, also today l connected 1k resistors to the gate of mosfets.
mosfets connected to pins 8 and 13 never switch ON. other two mosfets used to switch ON.
Thank you so much sir. Sir my question is about the first design (IRS2453). Those it means the ic is manufactured with the bootstrap and death time circuitry, or we can still add them to the circuit? Thanks hope to hear from you soon
The bootstrapping is already added in the circuit through the relevant diode and the capacitor, however, the values of these parts may need some variations depending on the frequency specifications of the system…
OK thank you sir like in my case the frequency is 50 to 60HZ and my DC supply is from a 48v serially connected battery instead of the rectified 220v ac than from there my output AC will be fered to the primary of a step up transformer to get my 220v AC. I hope there shouldn’t be any changes with the circuit. Thanks in advance
Yes, no serious changes required, except the bootstrapping capacitors which could be increased a bit until the most optimal MOSFET conduction and power output from the transformer is achieved
Am so greatfull sir but still am confused when you say “the value of the bootstrapping capicitors could be increased” Do you mean the capacitance or voltage rating of the Capacitors?, or are the capacitors non polarized type? Please sir can you kindly propose the capacitor values for me?. And lastly how can I provide a feedback circuit for my 220v stabilization? Thanks so much sir mey the lord be your strength ????.
It is the capacitance that may need to be increased, not the voltage rating. You can refer to the following diagram for the values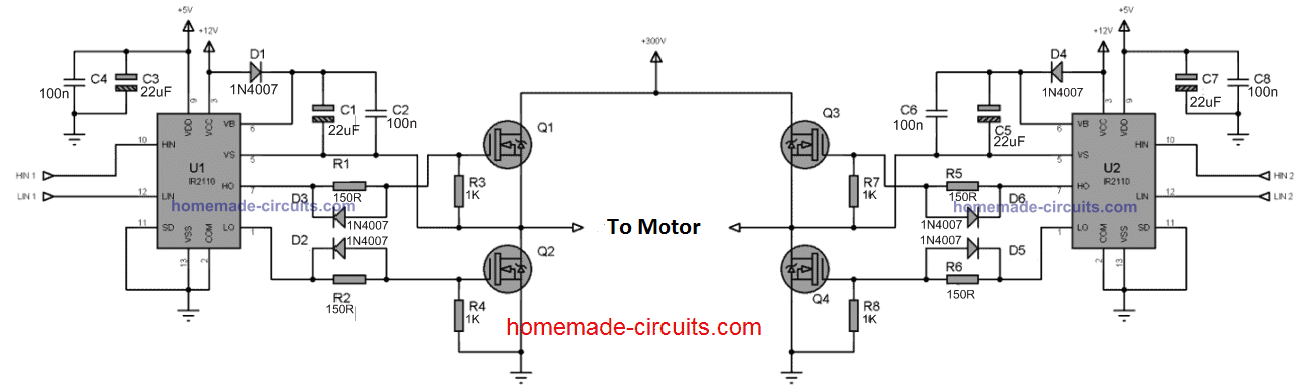
Once you complete it successful we can discuss the feedback.
Sir I need a complete guidelines on inverter design from scratch typically pure sine wave inverter using iron steel core transformer
George, You can read the following articles for the required information
Inverter tutorial
convert square to sine
Hi Swagatam, hope you well.
Hope you can help me with an idea i have with this named IC, called IRS2453(1)D.
Firstly, what is the chances to use two Hall effect transistors to do the timing of the IC? with other words, I have a rotor with say 10 small button magnets on, alternating between north and south. Then, using latching Hall effect tr’s which get switched on and off my the north and south magnets. then to use that switching sequence as the CT and RT timing for the said IC.
Secondly, my need is to use 2 of the said IC’s as to control a type of motor with the following working condition. the motor has 4 sets of electro-magnets and sits at the following positions, one at twelve o’clock and six o’clock and then the other two at three o’clock and then obviously at nine o’clock. The 12 o’clock and 6 o’clock works together and then obviously the other 2 works together, but with the distinct difference that at a one moment the 12 o’clock electro-magnet is a north pole and the 6 o’clock is a south pole. Then the next moment the sequence is reversed and the 12 o’clock electro-magnet has a south pole and the 6 o’clock has a north pole. Obviously the other pair works in the same manner due to the full H-bridge sequence and topology.
It has to be understand that when the one set op electro-magnet poles are sw’ed on the other pair has to be off. There has to be a certain on time and off time as to push and pull the magnets on the rotor to be moved. For better understanding of the motor, the rotor has 8 magnets which means at one moment each of the electro-magnets has 2 magnets, one north and one south. Thus when say the 12 o’clock eletro-magnet is sw’d on and forms a north pole, the it will push the north pole magnet on the rother and obviously pulls the south pole magnet. again it will be understood that the opposite electro-magnet will form a south pole and here again the logic is the same by the fact that the south pole magnet on the rotor will be pushed and the north pole magnet will be attracted. It will be then understood that this is the sequence of the other pole pair as well.
Here is where my first question came up as to see if i can use button magnets on a smaller rotor which is spaced accordingly as to sw on and off the hall effect tr’s as to cause the timing of the 2 H-bridge IC’s, causing the correct timing of the IC’s. It must beobvious to you now that i need to have a perfect sequence between the to timing H-bridge IC’s.
In fact Swagatam, this is a simple sequence when one uses a commutator, but its not the technology i want to use, but rather a electronical way and this said IC’s working is so simple that I am sure there must be a simple way to do it. My experience is 2-way radio’s and the repair of it and not in design of circuits as you are experienced in. Thus my reaching out to you and see if you might be able and eager to help as i get the idea when i went threw your web site that you enjoy designing and thinking outside the box.
So my friend, hope this i tried to explain to you is understanding and that it might inspire your to the point of thinking about it and design if necessary to help me in my little project.
Hope to hear from you and have a great day!
Regards
Ben Burger
Thank you Ben, I have understood your requirement, but I am having difficulty figuring out how the 4 sets of magnets can be switched with an H-bridge configuration.
It may be possible using a sequential IC such as a 4017, but not with an H-bridge, according to me.
Hi Swagatam, thank you for replaying…sorry my friend, I tried to explain the motor by saying there is 4 electro magnets where in fact there is 8. The purpose was to make it as plain as possible as to ensure you get the idea i tried to explain by using the analogy of the clock and the position of the electro magnets on the clock. There is in fact 2 electro magnets next to each other with a bit of a gap between them and the next 2 electro magnets. Its then also important to know that with 2 sets of electro magnets….which is directly opposing each other, there is 3 magnets for each set of electro magnets, which interact with the said electro magnets. It must be understood that when this two sets of electro magnets are active, on eletro magnet has a north pole and the adjacent electro magnet is then a south pole. At this specific moment of operation, with the one electro magnet a south and the adjacent eletro magnet a north, it causes the 3 permanent magnets to be pushed and pulled. It should be obvious that the operation of the opposite set of active electromagnets works exactly the same at the given moment. At this precise moment, the other two sets of electromagnets which is inactive, has each 2 permanent magnets right across it. With the rotor which is turning a few degrees the inactive electro magnets gets turned on and thus push the PM away as well, adding to the torque.
I hope I made the operation a bit more understandable.
I have considered the option of using a sequencer but came across this H-bridge IC and was wondering if it might work better.
I don’t want to put the motor diagram on this forum but if you like, as to understand this motor’s operation i will send you a sketch of it to your personal email address.
hope to hear again from you.
Friendly regards
Ben
Thank you Ben, I have already understood your explanation, and therefore I said 4 sets of electromagnets which corresponds to 8 electromagnets, where each pair is connected opposite to one another.
H bridge cannot be used here, because H-bridge can be triggered only through 2 complementary pulses, for operating a single flip-flop load.
If you are having other alternatives for operating this set up, then you can surely try them, because a Full bridge or an H bridge does not appear to be feasible for this application.
Hi Swagatam, thank you for your replay and have a great day.
Regards
Ben
You are welcome Ben….